Yale-led research models cell movements
The team has modeled cell mass movements, a challenging undertaking due to the complex nature of cell-to-cell interactions.
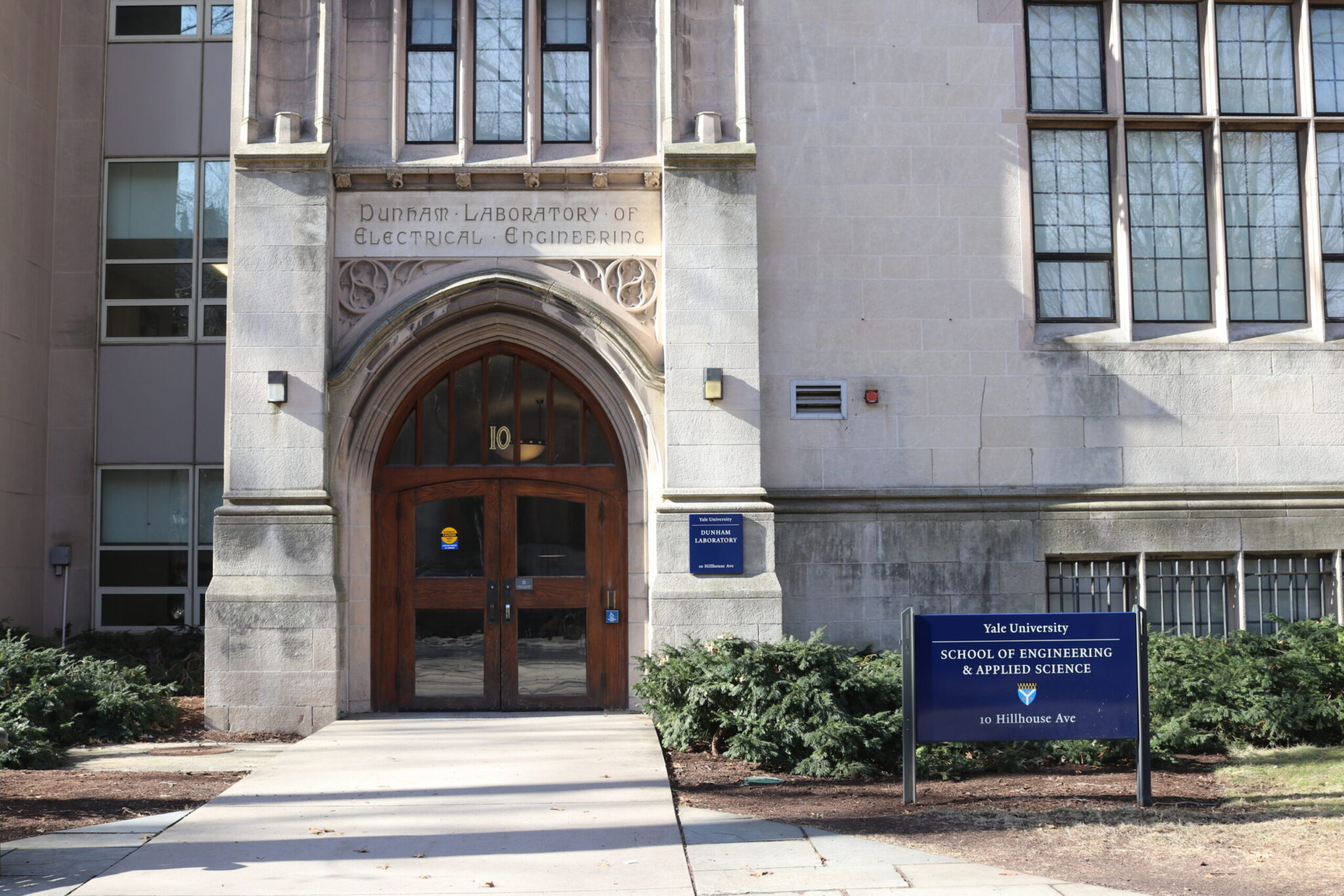
Kai Nip
A recent study from the School of Engineering & Applied Science took a key step in breaking down the complex, and often misunderstood, patterns behind how cells move through the body.
Published in the journal PRX Life and led by Yimin Luo, a professor of mechanical engineering and materials science, the project built a model to describe how cells move together in large groups. Since cells can bend and deform in unusual ways, researchers say, it can be difficult to predict how cells interact with each other and move en masse.
“We noticed that cells tend to align with density when we include more cells in our experiment … [which] made us realize that cell-to-cell interactions exist in our system,” said Xinyi Fang, a doctoral student at the University of California, Santa Barbara who co-authored the paper. “We wanted to construct a model that included both cell-to-cell interaction and also random movement.”
Cells are categorized as a type of active matter — matter that is composed of individuals that interact with each other but ultimately move in unison, said Luo. The term can refer to animal movement, like flocks of birds and schools of fish, and accurate models of bird and fish movements already exist.
However, the same models cannot be applied to cells, which deform and bend in unusual ways that disrupt traditional models.
In the process of designing a new model to address those shortcomings, the team first attempted to use conventional approaches to modeling active matter systems, said Mengyang Gu, a professor of statistics and applied probability at UCSB.
Their first attempt used a bottom-up model, which attempts to add up all forces occurring in the active matter system. To determine the next position for each cell, researchers would have to know every force acting on a cell at all times. But according to Luo, that process is impractical, since cell movements are prohibitively complex to predict.
A more accurate model of cell movement, they hypothesized, would contain some level of randomness.
The second method attempted to use an artificial intelligence model to track patterns in active matter, said Luo. After being trained on sample datasets of cell movement, the AI would try to determine a pattern to predict how individual cells in groups move.
But, by itself, the AI did not work for cells either. Luo said that there was too much fluctuation in cell movements, and cells move too slowly — factors that introduce too much noise into the system for the model to predict.
Instead, the team opted to use a hybrid of the two models. The team used elements of the bottom-up model to reduce the noise in the training dataset and clarify peculiarities in how cells interact.
For example, when cells move in opposite directions, they slide over each other instead of bouncing off of one another and changing their direction — a case that would confuse the AI model. After factoring in those special cases, the team used AI to model interactions afterward.
According to the researchers, a better understanding of cell dynamics can have wide-reaching impacts.
For Fang, the research can provide key insights into the way that wounds heal. The model can be applied to the way that cells migrate to the area of a wound and start to repair the damaged tissue.
Luo also believes that his team’s research could help scientists better understand cancer. Most cancer patients who succumb to the disease, Luo said, die from cancer metastasis — the movement of cancer cells from the primary tumor to other parts of the body. The researchers hope their model can advance scientists’ understanding of how those cancer cells move throughout the body.
“Most of this would be applied or would be applicable to cases of cell migration, which is implicated from anywhere like embryonic development to cancer metastasis,” said Luo.
Gu also anticipates the model to be useful in the creation of soft robotics — a field that includes the creation of artificial cells. According to Gu, this model may aid in that endeavor. But to do so, the researchers hope to make their model more representative of real-life cells.
While the study only models cells in two dimensions, there aren’t a lot of cell interactions in the body that can be accurately described with 2D-movement, Luo added. Only bodily systems like skin or the eyes’ external layer of protective cells can be realistically approximated to a 2D plane.
“The next step would be taking this to 3D,” said Luo. “There are challenges that we have to overcome, but the 3D model would be more realistic than the 2D model.”
Expanding the model to predict cell movements in three dimensions, however, is not a simple undertaking. According to Luo, one of the biggest obstacles is the challenge of imaging 3D cell movements.
As it stands, Imaging a 2D system is relatively easy: researchers can illuminate and photograph cells moving. However, illuminating a 3D system would cause lights to bounce off of the various elements in the system, reducing a scan’s resolution to an unusable degree.
The problem of resolution is an important next step in the creation of a more comprehensive 3D model, the researchers said.
“I think understanding how cells interact is important for many,” said Gu. “Understanding how they walk, how they interact, is the first step to develop many new applications and materials.”
The School of Engineering & Applied Science is located at 17 Hillhouse Ave.