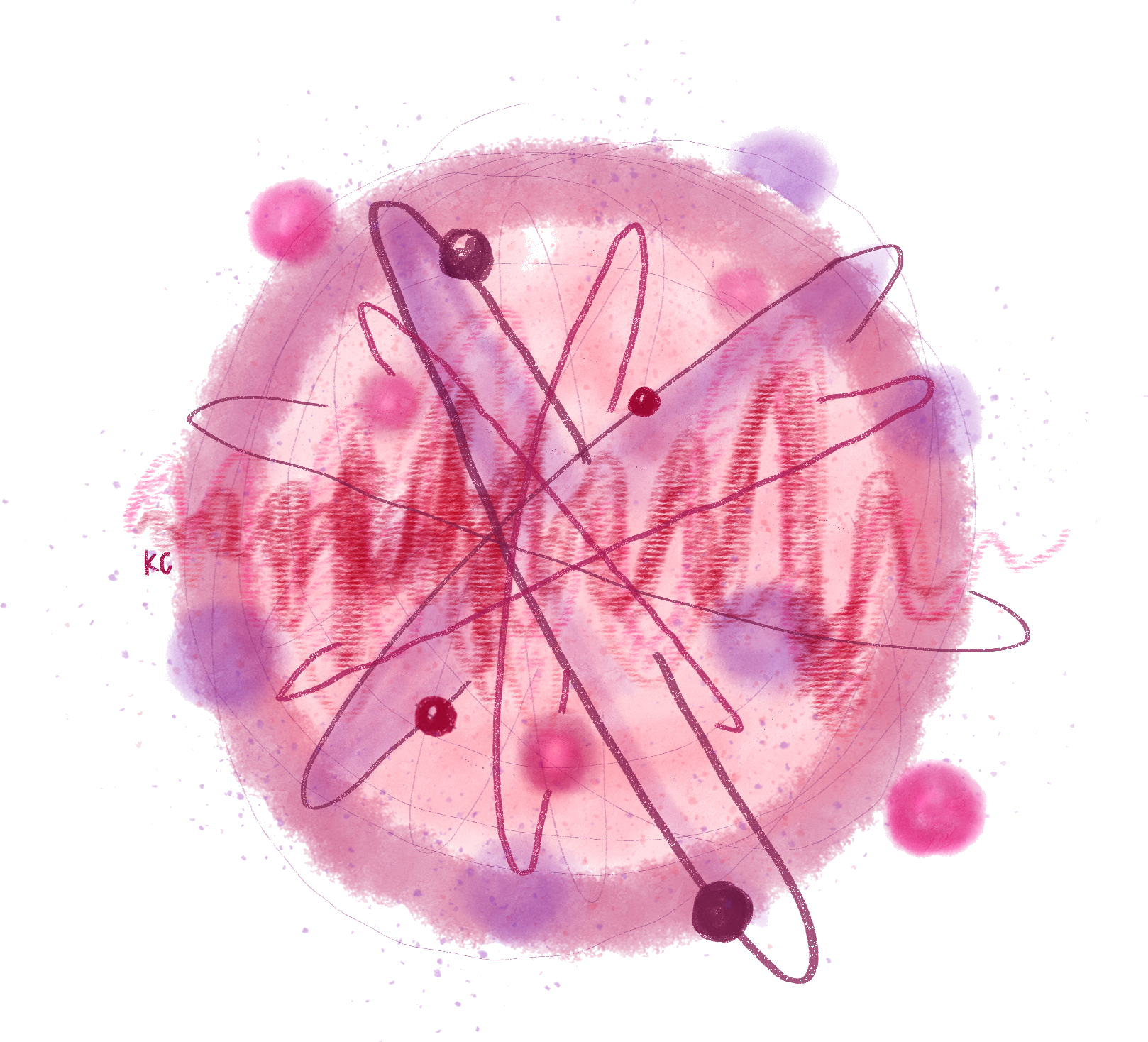
For decades, physicists have searched for a theory to describe how all of the universe works. But a new collaboration between Harvard and Yale may have debunked some of the main theories physicists have been cultivating for the past 30 years.
Researchers in the Advanced Cold Molecule Electron Electric Dipole Moment Search — which includes Yale physics professor David DeMille and his team of graduate students and postdoctoral associates — have been searching at an extremely precise scale for the electron’s electric dipole moment, a little bulge that would indicate that its charge is not in the shape of a perfect sphere. This bulge would be caused by an unknown heavy particle, whose existence would help explain many of the universe’s unsolved mysteries.
“Our results and results from experiments like the Large Hadron Collider and dark matter experiments are looking for mass at this range,” said DeMille. “Certain things about the universe would be much easier to explain if we had really big particles. Since we didn’t see the [electric dipole moment], or evidence of the existence of really big particles at this scale, most of the current predictions of theorists look like they’re not true.”
Currently, physicists use the Standard Model — a theory of how all particles and matter interact with each other — to describe how the universe works. But the Standard Model does not explain every aspect of the universe, a fact well known in the world of physics.
According to DeMille, the two big unexplained mysteries of the universe are dark matter and antimatter. Dark matter is an invisible, unknown, matter-like substance that explains why galaxies move faster than they should, considering the amount of mass they contain. Antimatter is matter that, mathematically, moves backwards in time; whenever energy is turned into mass, antimatter and matter are created in equal amounts. Though physicists understand the concepts of dark matter and antimatter, they do not know why there is so much matter, and so little antimatter, in our universe.
“The energy in the Big Bang should have done the same thing, but there’s basically no antimatter left in the universe, compared to the amount of matter,” DeMille said. “When you compare the total matter in the universe to the total energy, one billionth of the expected matter still exists. The rest apparently turned back into energy by matter and antimatter combining. How did it happen that matter remained?”
DeMille has been working to find the electron’s electric dipole moment since he was a postdoctoral associate at the University of California, Berkeley, 25 years ago. When he moved to Yale, he started a similar experiment in the basement of Sloane Physics Laboratory. After 10 years of minimal progress, he heard of a new technique made by Harvard professor John Doyle, one of DeMille’s collaborators at the time. Along with former Harvard physics professor and current director of the Center for Fundamental Physics at Northwestern University, Gerald Gabrielse, who was also curious about the new technique, the three decided to start a research group to search for the dipole moment.
The team’s process included putting an electron inside a molecule of thorium oxide, which has one of the highest electric fields of all chemical compounds. This high electric field within the molecule made the electron spin in a certain direction. After the molecule moved, the physicists used lasers to measure whether or not the direction had changed. A change in direction would indicate that an electric dipole moment exists. The team did not detect an electric dipole moment, a sharp contrast to what modern theories suggest.
Xing Wu, a postdoctoral associate in DeMille’s lab, noted that they used a “blind analysis” approach: having the group predict what certain outcomes would mean before actually seeing the data. When the data was finally revealed, this approach led to a “ceremony,” Wu said.
“In the end, I believe our ‘unblinding’ made some people a little disappointed, since we measured an EDM value still consistent with zero, but at the highest ever precision,” Wu said. “Nevertheless, this null result is still quite exciting, and it sheds some light upon the unknown part of our universe.”
When describing the precision referenced, DeMille said that if an electron were the size of Earth, the width of the dipole that the collaboration is searching for would be 20 nanometers, or one quadrillionth of its radius.
DeMille noted that the public can be disappointed when an experiment keeps seeing zero. But he praised the National Science Foundation, which funds his experiment, for being “brave in the face of public pressure,” and continuing to fund projects over the long term. For example, he pointed to the Laser Interferometer Gravitational-Wave Observatory, which received NSF funding for several decades with few results before finally finding evidence of gravitational waves in 2016.
The Advanced Cold Molecule Electron Electric Dipole Moment Search is not the only group searching for evidence of a new theory of the universe. In a 16-mile circular tunnel underneath Geneva, Switzerland, the Large Hadron Collider accelerates small particles such as electrons or protons to almost match the speed of light. By colliding these high-speed particles at the right speed, the desired heavy particles could potentially be created. Though researchers working with the Large Hadron Collider have not yet found these massive, unknown particles, they could create them with a new, bigger collider if the Yale-Harvard collaboration succeeds.
“[The Yale and Harvard team] found so little of an effect that they ruled out particles of an energy that [the Large Hadron Collider] could find,” said Steve Girvin, Yale physics professor and former deputy provost for science and technology. “It’s incredible. With an experiment the size of practically a tabletop, or half a room, they can compete with the [Large Hadron Collider] with looking for these particles. It’s a pretty big deal.”
Though his collaboration has not found evidence of the electron’s electric dipole moment yet, DeMille said that they can reach an even greater precision in the future, hopefully finding evidence of an even more massive particle than what the precision they have used so far has allowed.
DeMille noted that by eliminating noise in their data or using a technique in which lasers suspend a molecule for as long as a second, the research team could possibly still see evidence of a bigger particle.
“There’s a lot of excitement in the field,” said DeMille. “There’s a real chance to discover something even when us old folks are alive. There are absolutely no guarantees, but it’s possible … As long as we keep improving rapidly, it’s easy to keep up the enthusiasm. If nature is kind, we will be able to find new particles.”
The Higgs Boson, another particle previously predicted by particle physicists, was formally found using the Large Hadron Collider in 2012.
Helena Lyng-Olsen | helena.lyng-olsen@yale.edu .